Gravitational Waves & Universe Sandbox ²
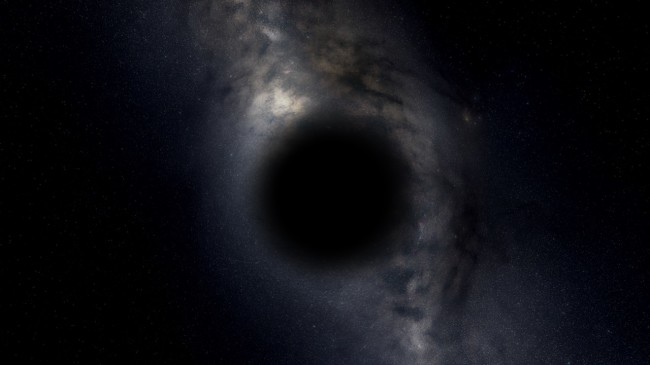
A black hole in Universe Sandbox ². Researchers concluded the detected gravitational waves resulted from two black holes colliding.
What’s the significance of discovering gravitational waves?
This announcement is a huge deal. It is on par with the discovery of the Higgs Boson particle which provided the missing evidence for a prediction of the Standard Model of particle physics. Gravitational waves are a century-old (almost exactly) prediction now confirmed by a huge number of relentless, and brilliant people after many years of hard work. It is the first direct confirmation of the prediction from Einstein’s General Relativity that matter and energy determine the motion of bodies by warping the fabric of spacetime itself, and in so doing, emanate ripples when massive bodies are accelerated through that space.
It is not only confirmation of general relativity, though. It is also the first of many future observations that will look at the universe in a completely new way. Up until now we’ve used only photons (telescopes all along the electromagnetic spectrum) and sometimes neutrinos. Now we can add listening to the fabric of space to our list of tools. This will allow us to see the dark and the obscured parts of the universe: the early universe, centers of galaxies, things blocked by dust clouds, and so on, by listening for changes in space itself. It is the start of a new age in astronomy.
In addition to this detection being the first direct proof that the predictions of general relativity that matter and energy warp space time are true, and some of the strongest evidence for the reality of black holes, this is also a new kind of astronomy. Though gravity is the weakest force and gravitational waves are very hard to detect, they do have a few advantages over observations of photons.
- First, gravitational waves are practically impervious to matter in their path. This means we can see into regions of space that are blocked to optical observatories, such as inside dense clouds of dust, the centers of galaxies, behind large or close bodies.
- Second, this is an observation of the warping of space itself, meaning we can detect things that have mass but might not produce observable light, such as black holes, dense sources of dark matter (if such were to exist), cosmic string breaks, etc.
- Third, gravitational waves fall off in amplitude much more slowly than light. This means that we can receive signals from very far away that we might not notice optically.
- And fourth, because gravitational waves also travel at the speed of light and don’t have to bounce off intervening matter, and begin to be potentially detectable from bodies getting close rather than just after the moment of collision, this means that we can work with other telescopes and tell them “Look over there! You’re probably going to see something exciting!”
This all of means that this detection means the beginning of a new kind of observational astronomy, as well as a better understanding of of of the fundamental forces of the universe, gravity.
What role did Jenn, astrophysicist and Universe Sandbox ² developer, play in the discovery?
While I was in the field I ran super-computer simulations to make predictions about the gravitational wave signals that would be produced by binary black hole mergers. Those waveforms are used as templates in the detector pipeline. The detector matches the template banks against the incoming data to find real signals amidst the noise of the detector, while also doing searches for large burst signals (how this one was found). Those waveforms are then used again to determine where the signal came from, what it was (two black holes, a neutron star and a black hole, two neutron stars, etc), and the properties of the bodies that created the signal (spins, masses, separation, etc.). I also worked on developing the analytical formulas to determine those spins and masses from those signals.
Here’s one of the scientific papers on the process of determining the properties of the source of the signal, with three papers cited on which Jenn Seiler was an author:
https://dcc.ligo.org/public/0122/P1500218/012/GW150914_parameter_estimation_v13.pdf
The Einstein equations for general relativity are ten highly non-linear partial differential equations. This means that it is only possible to obtain exact solutions for astrophysical situations for some very idealized conditions (such as spherical symmetry and a single body). In order to predict the gravitational waveforms produced by compact multi-body systems, or stellar collapse, it is necessary to solve the equations numerically (computationally). This means formulating initial data for spacetimes of interest (such as two in-spiralling black holes of various spins and mass ratios) and evolving them by integrating the solutions of the Einstein equations stepping forward in time by discrete steps. To prove that these computer simulations approximate reality more than just by equations on paper we would run these simulations at multiple resolutions for our discrete spacetimes and show that our solutions converged to a single solution as we approach infinite resolution (that would represent real continuous space) at the rate we expect for the method we were using.
There were many obstacles in creating these simulations: vast amounts of computational power required for accuracy; the fact that we needed to run tons of these large, slow, computationally intensive simulations in order to cover the parameter space (spins, masses, orientations, etc) of potential sources of gravitational waves; and so on. For black holes, one major challenge was the fact that they contain a singularity. A singularity means an infinity, and computers don’t like to simulate infinities. Numerical relativity researchers had to find a way to simulate black holes without having the singularity point in the slicing of the spacetime integrated in the simulation. The first successful simulation of this kind didn’t happen until 2005 (http://journals.aps.org/prl/abstract/10.1103/PhysRevLett.95.121101).
Once we had working simulations, groups around the world set to work on simulating the gamut of major potential gravitational wave signal sources. These simulation results were not just useful to the detectors to help identify signals, but also to the theorists to help formulate predictions about the results of such astrophysical events. Predictions such as: the resulting velocity of merged black holes from binaries of various spins, the amount of energy released by black hole mergers, the effect that black hole spins have on the spins and orbits of other bodies, etc.
When will you add gravitational waves into Universe Sandbox ²?
We really can’t do gravitational waves in an n-body simulation, which is the method Universe Sandbox ² uses to simulate gravity. N-body simulations look at the effect that each body has on each other body in a system at small discrete time steps.
General relativity requires simulating the spacetime itself. That is, taking your simulation space, discretizing it to a hi-res 3-D grid and checking the effect that each and every point in that grid has on all neighboring points at every timestep. Instead of simulating N number of bodies, you are simulating a huge number of points. You start with some initial data of the shape of your spacetime and then see how it evolves according to the Einstein equations, which are 10 highly non-linear partial differential equations. Accurate general relativity simulations require supercomputers.
There are some effects and features related to relativity that would be possible to add to Universe Sandbox ², however. Here are a few we are discussing:
-
Gravity travelling at the speed of light.
-
Currently if you delete a body in a simulation, the paths of all other bodies instantly respond to the change. The reality is that it would not be instantaneous; it would take time for that information about the altered gravitational landscape to reach a distant object.
-
-
Spinning black holes.
-
Most black holes are very highly spinning. If you imagine a spinning star collapsing it is easy to understand why. This is the same effect as when a spinning figure skater pulls in their arms; because of conservation of angular momentum, they spin faster. A consequence of this spin is that, while the event horizon would remain spherical, there would be an oblate spheroid (squished ball) around the black hole called an ergosphere. This ergosphere twists up the spacetime contained within it and accelerates bodies that enter this region (as well as affecting their spins). Because it is outside of the event horizon, this means one can slingshot away from this region and even steal energy from the rotation of that black hole.
-
-
Corrections to the motions of bodies to approximate general relativity.
-
Loss of momentum due to the emission of gravitational waves causes close massive bodies to inspiral. With this you could recreate the decaying orbits of binary pulsars.
-
Spins of close bodies affect each other’s motion and spins (see above). This would give you things like spun up accretion disks around black holes.
-
These corrections would be made by adding post-newtonian corrections to body velocities.
-
Learn more
Bad Astronomy article: LIGO Sees First Ever Gravitational Waves as Two Black Holes Eat Each Other
Video and Comic Explaining Gravitational Waves
Reddit AMA (Ask Me Anything) by LIGO Scientists
Paper by LIGO Researchers: Observation of Gravitational Waves from a Binary Black Hole Merger
about 9 years ago
there is a problem when you dosen t have sun (I mean in that system)planet or moon is half still have ligth of sun or other stars why it not gone? or I found a BUG ? pleash chach it
about 9 years ago
It’s a tricky situation because most users still want to be able to see the objects in their simulation, even if there is no star. But maybe there should be an option for turning off the phantom light.
about 9 years ago
I’m pretty sure there is a way to turn it off
about 9 years ago
You can change the lighting mode in View>Light, but there will still be a light source even in sims without a star.